1. Multiscale characterization of left ventricle active behavior in the mouse.
The myocardium possesses an intricately designed microarchitecture to produce an optimal cardiac contraction. The contractile behavior of the heart is generated at the sarcomere level and travels across several length scales to manifest as the systolic function at the organ level. While passive myocardial behavior has been studied extensively, the translation of active tension produced at the fiber level to the organ-level function is not well understood. Alterations in cardiac systolic function are often key sequelae in structural heart diseases, such as myocardial infarction and systolic heart failure; thus, characterization of the contractile behavior of the heart across multiple length scales is essential to improve our understanding of mechanisms collectively leading to depressed systolic function.
In this project, we developed a novel methodology to characterize the active behavior of left ventricle free wall (LVFW) myocardial tissues in mice. Combined with active tests in papillary muscle fibers and conventional in-vivo contractility measurement at the organ level in an animal-specific manner, we established a multiscale active characterization of the heart from fiber to organ. In addition, we quantified myocardial architecture from histology to shed light on the directionality of the contractility at the tissue level. This multiscale experimental pipeline is expected to provide crucial insight into the contractile adaptation mechanisms of the heart with impaired systolic function.
2.Adaptive architectural remodeling of the RV in PH
In patients with pulmonary hypertension (PH), the right ventricle (RV) experiences a severe pressure overload and undergoes extensive remodeling at the fiber, tissue, and organ levels. Despite significant progress in characterizing the global and functional behavior of the RV in healthy and PH subjects, our understanding of fiber- and tissue-level remodeling events leading to RV functional adaptation remains limited. Also, the technology to discern and quantify these events in vivo remain elusive.
In this project, we developed a high-fidelity computational cardiac model of sugen-hypoxia rodents, capturing the relationship between RV architecture obtained from diffusion tensor imaging and RV function . This model will allow us to study the capacity of architectural remodeling to maintain ventricle-arterial coupling and lead to RV functional adaptation.
Normal Heart Hypertensive Heart
3. Left ventricular remodeling in myocardial infarction
Myocardial infarction (MI) results in cardiac myocyte death and the formation of a fibrotic scar in the left ventricular free wall (LVFW). In the days and weeks that follow an acute MI, left ventricular (LV) remodeling, which consists of several alterations in the structure and properties of cellular and extracellular components of the LVFW, becomes an important predictor of clinical outcomes. The normal function of a heart is strongly influenced by the passive biomechanical behavior of the LVFW, and progressive myocardial structural remodeling can have a detrimental effect on both diastolic and systolic function of the LV leading to heart failure.
3.1 Characterizing region-specific remodeling of left ventricular myocardium in MI
In this project, we study the LVFW remodeling in the rodent model of MI induced by ligation. Our goal is to investigate the multiscale aspects of the LVFW remodeling and separate the contributions from underlying fiber-level remodeling events to changes in tissue-level mechanical behavior. These events include time-course structural and compositional alterations of collagen network and myofibers and exhibits regional heterogeneities in the LVFW. The identification of these events and characterization of their time-varying effects on tissue-level behavior provides important mechanistic markers incremental to global indices for improved prognosis in MI.
3.2 Structural determinants of left ventricle function in MI
In this project, we use an integrated high-fidelity computational cardiac models of the infarcted heart in rodent models of MI to study the effect of longitudinal changes in mechanical and structural properties of the LVFW on left ventricle function. Ultimately, the subject-specific understanding of the remodeling state post-MI, with a focus on regional biomechanical and contractile changes, will enhance current prognostic tools and provides an in-silico platform to develop and evaluate patient-specific mechanical therapies for MI.
4. Non-rigid image registration using cardiac magnetic resonance imaging
Accurate assessment of cardiac contractile function is crucial to improving the mortality associated with adverse cardiac events. Spatial heterogeneity is a feature of many pathologies which impair left ventricle (LV) contractile function. Global biomarkers of contractile function, such as ejection fraction, overlook regional variations in contractile function and do not provide insight into the underlying mechanisms. Therefore, there is a need for mechanistic tissue-level biomarkers.
Cardiac magnetic resonance imaging (CMR) provide cine cardiac images using FLASH sequence. In this project, we used non-rigid image registration technique and FALSH scans to quantify four-dimensional cardiac motion in terms of 3D cardiac strains versus time. Impairments and adaptations of cardiac strains will serve as mechanistic biomarkers providing insights into regional passive and active adaptations prior to organ-level functional manifestations. We are currently exploring these markers in mice with heart failure with preserved ejection fraction.
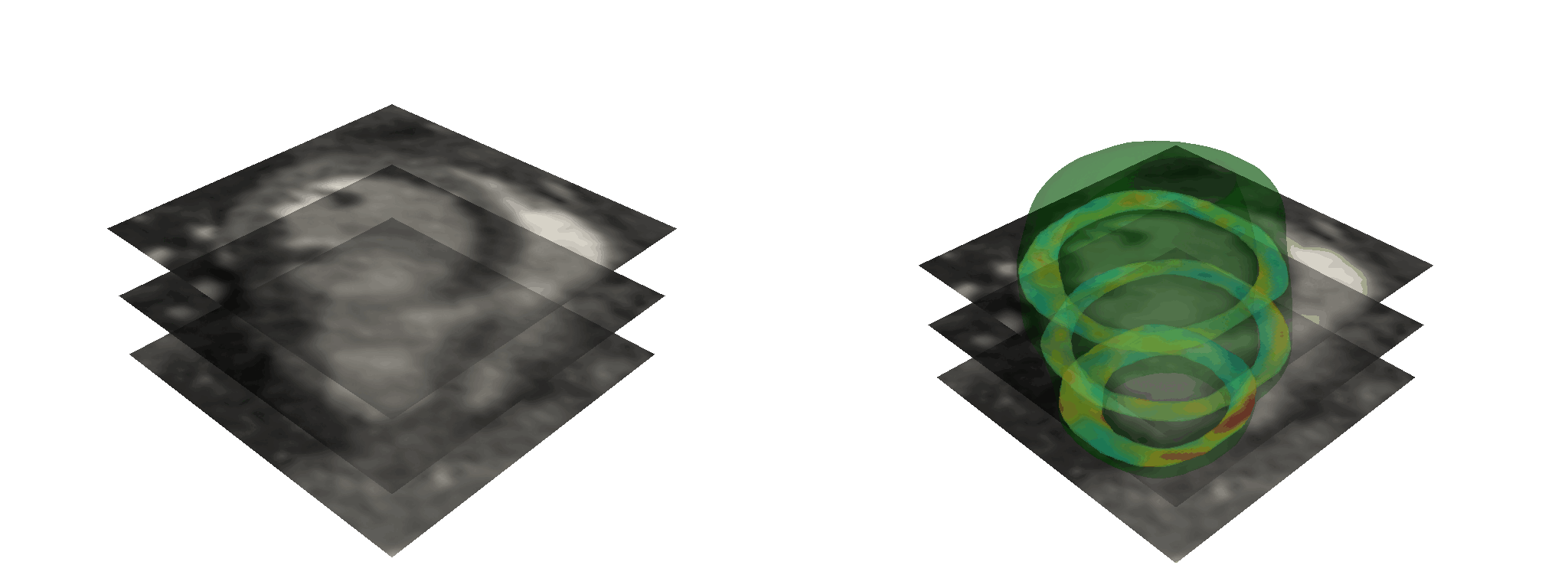
CMR stack and strain from image registration
5. Integrated echocardiography –biomechanics approach to study HFpEF
Heart Failure with preserved ejection fraction (HFpEF) accounts for over half of all heart failures in the US. A major feature of HFpEF is left ventricular diastolic dysfunction (LVDD). The causes of LVDD remain varied and current diagnostics (limited to organ level measurements) cannot differentiate pathophysiological heterogeneity. The development of non-invasive techniques is vital to advance our understanding of HFpEF. An integrated echocardiography-biomechanics approach was used to study characterize myocardial remodeling.
A speckle tracking echocardiography (STE) was used to quantify cardiac strains in a murine model of HFpEF and control mice. Our STE package uses 2D ultrasound B-mode images to generate standard cardiac strains in AHA segmentation format. Lagrangian strain and strain rates (radial, circumferential and longitudinal) were calculated at end diastole relative to end systole to assess filling abnormalities at the tissue level. Mouse-specific myocardial specimens were harvested after ultrasounds scanning and underwent mechanical and structural characterizations. Our results suggest that echocardiography can be used to assess intrinsic myocardial stiffening in HFpEF.
6. A machine learning model to estimate myocardial stiffness from EDPVR
In-vivo estimation of mechanical properties of the myocardium is essential for patient-specific diagnosis and prognosis of cardiac disease involving myocardial remodeling, including myocardial infarction and heart failure with preserved ejection fraction. Current approaches use time-consuming finite-element (FE) inverse methods that involve reconstructing and meshing the heart geometry, imposing measured loading, and conducting computationally expensive iterative FE simulations.
In this project, we develop a machine learning (ML) model that feasibly and accurately predicts myocardial properties directly from select geometric, architectural, and hemodynamic measures, thus bypassing exhaustive steps commonly required in cardiac FE inverse problems. Geometric and fiber-orientation features were chosen to be readily obtainable from standard cardiac imaging protocols. A multi-layer feed-forward neural network (MFNN) was used as a deep learning agent to train the ML The trained ML model offers a feasible technology to estimate patient-specific myocardial properties, thus, bridging the gap between end-diastolic pressure-volume relationship (EDPVR), as a confounded organ-level metric for tissue stiffness, and intrinsic tissue-level properties. These properties provide incremental information relative to traditional organ-level indices for cardiac function, improving the clinical assessment and prognosis of cardiac diseases.
7. Computational modeling of the lung to study the effect of regional inflammation on lung function
Lung biomechanics is an active field of study that aims to understand the relationship between structure and function in the lung under normal and pathological conditions. Many pathological conditions—including acute respiratory distress syndrome (ARDS), emphysema, and idiopathic pulmonary fibrosis—alter the structure of the lung in acutely or in a delayed manner leading to lung functional decompensation. The importance of understanding lung biomechanics is clear, especially in light of the COVID-19 pandemic.
Computational modeling of the lungs is an active field of study that integrates computational advances with lung biomechanics, physiology, and medical imaging to promote individualized diagnosis, prognosis, and therapy evaluation in lung diseases. Alveolar surface tension caused by the liquid film lining the alveoli and regulated by surfactant concentration is a key aspect of respiration. The disruptions in normal surface tension due to lung inflammation easily translate to respiratory dysfunction.
In this project, we use an image-based lung model and develop a material behavior that addresses the effect of varying surface tension during respiration. The material behavior is developed for a single alveolus and scaled up to the bulk tissue. The model can then account for the effect of fluid build-up in the alveoli to simulate the effect of regional and heterogenous inflammation on lung function.